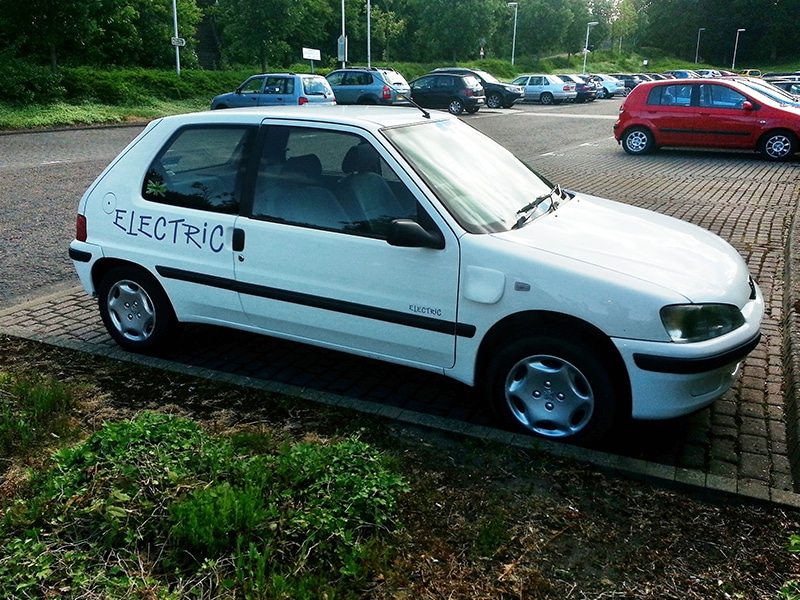
The Peugeot 106 EV
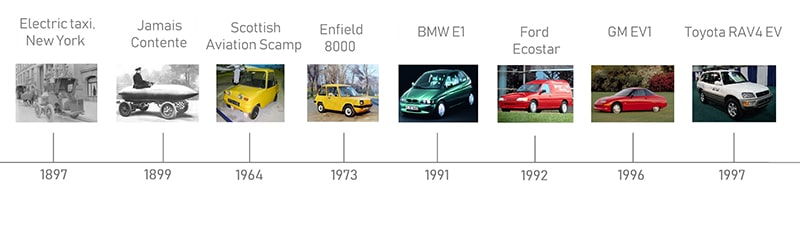
When many people think of the first electric vehicle on the market, they may think of the Nissan LEAF, the Tesla Roadster or even the bijou town car and mainstay of EV photos on BBC News articles, the Reva G-Wiz. However, the dawn of the EV dates back to the dawn of the car, when Scottish inventor Robert Anderson created the first electric carriage in the 1830s. This car was so visionary that it predated rechargeable batteries, so was powered using a primary (single-use) “voltaic pile” that required replacement once it had run flat. The invention of the lead-acid battery by Gaston Planté in 1859, and its refinement by Camille Alphonse Faure in 1881, finally gave the burgeoning automotive industry a secondary (rechargeable) cell that would not require replacement after every discharge.
By the end of the 19th century, EVs had a sizeable market share in comparison to internal combustion engines (ICEs) and steam-powered cars. They proved to be particularly popular in urban areas – notably amongst women drivers – on account of being easy to drive and recharge, quiet, emissions- free and much safer to use than an ICE, which had to be started using a dangerous and occasionally bone-shattering crank handle. New York had a fleet of electric taxis that were kept going by battery swapping stations, well over a century before Renault and Tesla experimented with the idea. In 1899, lead-acid batteries powered ‘Le Jamais Contente’ past the 100 km/h barrier, setting a world speed record in the process. However, lead-acid batteries were heavy; a prime example is the 500 kg Tudor battery pack which comprised 37% of the kerb weight of the Egger-Lohner C.2 Phaeton EV, which is technically the first Porsche ever made. This meant that any increase in top speed would come at the heavy sacrifice of range. For now, this wasn’t an issue since roads connecting towns and cities were generally in poor condition, so speed and power demand were typically low, allowing some early EVs to achieve 100 miles on a single charge.
The first genuine competitor to lead-acid batteries arrived at the turn of the 20th century in the form of Waldemar Jungner and Thomas Edison’s nickel-iron battery. Tolerant of over-charge, over-discharge and long-term storage, and boasting improved energy density and a lifespan of several decades if properly maintained, nickel-iron batteries were soon offered as optional, but expensive, upgrades for some electric vehicles, such as the Detroit Electric (several of which, incidentally, were owned by Henry and Clara Ford). However, nickel-iron cells have low coulombic efficiency (energy in vs energy out) – as little as 50% in some cases – and a high rate of self-discharge, with charge depleting by 15% per week even if the battery isn’t used. As road quality improved, speeds increased and the electric starter motor made ICEs much safer to use, battery development couldn’t keep pace with rival propulsion systems and electric vehicles were all but sidelined for most of the first half of the 20th century.
In the 1960s, Prestwick-based Scottish Aviation saw an opportunity to tackle congestion and pollution in urban areas and decided to create its own electric town car, the Scamp. Powered by lead-acid batteries, the compact machine had a mere 36 mph top speed and could manage just 18 miles per charge. However, Scottish Aviation had set their sights on a new family of cell chemistries that promised vast improvements in range over lead-acid batteries: “breathing” metal-air cells. These have the advantage of sourcing some of their reactants from the air rather than storing all chemicals within the cell, which not only makes them lighter and more compact, but also makes them safer. Scottish Aviation hoped to be able to use zinc-air cells in the Scamp within a few years; over half a century on, the only version of zinc-air cells that has been commercialised is a small, single-use variant used in hearing aids. Scottish Aviation cancelled the Scamp project after encountering reliability issues with the suspension during testing; twelve prototype vehicles had been built.
The oil crisis of the 1970s saw a return to EV research and development on both sides of the pond. In 1973, the Enfield 8000 was launched in England. This car has a claim to Fully Charged fame, presenter Jonny Smith converted one into the world’s fastest street legal electric drag racer. Needless to say that the original 6 kW motor and lead-acid battery pack were not used. Impressively, the diminutive motor could shift the car up to 48 miles per hour, with the lead-acid cells providing a range of 40 miles. Similar performance specs were offered by the Sebring-Vanguard CitiCar, which held the EV record for the greatest number of units built in the US (4,444) until this was surpassed by the Tesla Model S.
Governments’ increasing recognition of climate change, and desire to act, resulted in a raft of environmental legislation that forced automotive manufacturers to revisit electric vehicles in the late 80s and early 90s. This time, several new battery chemistries were available to try. Molten salt cells offered a four-fold increase in range vs lead-acid batteries, respectable coulombic efficiency of around 90% and a low rate of self-discharge, but vehicles that use them must be kept plugged in when not in use to keep the salts at temperatures of around 300 degrees C and stop them solidifying. Sodium- sulphur variants were used in the BMW E1 (which looks like an early 90s version of the i3) and the Ford Ecostar (essentially an electrified Escort van), giving the vehicles a range of 100 and 125 miles per charge, respectively. Unfortunately, molten sodium polysulfides are corrosive, and sodium- sulphur cells are highly reactive if the two reaction vessels come into contact. The Ford Ecostar suffered a couple of battery fires whilst charging, and the project was halted. A safer variant of molten salt cell, the ZEBRA cell, was trialed in the BMW E2, but BMW concluded that the need to spend up to 15% of the pack’s capacity per day just on keeping the salt molten meant that EVs were not ready for commercialisation. This didn’t deter Modec from offering ZEBRA cells in their large delivery vans in the mid-2000s.
The mid to late 1990s saw the arrival of a raft of new EVs in the US, powered by nickel metal hydride (NiMH) cells. These offered a four-fold increase in energy density vs lead-acid and a high power density, typically giving vehicles that used them a range of 100 miles per charge and the ability to be driven at highway speeds. General Motors’ EV1 is the most famous of this group of EVs, but arguably the most impressive was the Toyota RAV4 EV, which was unveiled in 1997. Like the EV1, the RAV4 EV was charged using a Magne Charge inductive paddle, an early form of wireless charging. Unlike the EV1, it was a full-size SUV with five passenger seats and ample luggage space. Unfortunately, the success of this highly practical EV was too much for oil giant Chevron to handle: it bought the patent for NiMH cells and sued Panasonic, the manufacturers of the EV-95 cell used in the RAV4 EV. Production of large-format NiMH cells was halted, relegating their production to small cells used in hybrid battery packs, and the EV revolution was delayed by about a decade. In contrast to the fate of the EV1, many original RAV4 EVs are still in use today, some using their original battery packs.
Around the same time, in Europe, PSA Peugeot-Citroen opted to trial nickel-cadmium cells. These cells have approximately double the energy density of lead-acid cells, but suffer from a “memory effect” whereby, if the cell is routinely discharged to roughly the same mid-point State of Charge rather than being fully discharged, the cell acts as if it is fully discharged when it actually has capacity remaining. The cells used by PSA also required periodic topping up with deionised water, performed via the car’s built-in plumbing. PSA produced 10,000 electrified versions of the 106, Saxo, Berlingo and Partner between 1995 and 2005, some of which made it to the UK. The Peugeot 106 Electric was a rare sight, but was found in local authority fleets in the likes of Newcastle, Coventry and Edinburgh, and was responsible for the installation of the first on-street charge point in the UK. It is also the first EV that I ever drove, and the one that inspired me to work on electric vehicles and battery tech (I didn’t choose the plug life; the plug life chose me). The 20 horsepower motor was surprisingly punchy around town and on country roads, and could power the car to an electronically-limited 60 mph, with the 12 kWh battery pack providing a range of 50 miles per charge. Unfortunately, PSA’s cell manufacturer realised too late that there was a defect in the separators in their cells, leading them to short circuit and die early. Furthermore, NiCd cells were later banned due to their use of toxic heavy metals and the availability of less toxic alternative chemistries. Many of PSA EVs were repatriated in a fate similar to the GM EV1, but some slipped the net – including my own. It is now on display in Dundee Museum of Transport next to a Scottish Aviation Scamp.
Despite first being commercialised by Sony back in the early 1990s, it would be nearly two decades before lithium-ion battery packs were first scaled up and used in mass-produced EVs, starting with the Tesla Roadster and Mitsubishi i-MiEV. High energy density and respectable power density were offset by expense and sensitivity to temperature, over-charge and over-discharge, which required advanced control electronics in the form of a battery management system (BMS). In the mid-to-late 2000s, a number of firms saw an opportunity to beat the big automotive manufacturers to market with lithium-ion powered EVs. In Scotland, Allied ZEV retrofitted ICE vans to electric drivetrains, using “prismatic” (chunky box style) cobalt-free lithium iron phosphate cells from China, which were assembled into battery packs by Axeon in Dundee. Coventry-based Modec offered the choice of molten salt and lithium-ion battery packs for its large delivery van, with Axeon assembling the latter. Smith Electric Vehicles also retrofitted ICEs to EV, with a range that included a 7.5 tonne truck. Unfortunately, all of these vehicles were too far ahead of their time; they predated modern charging infrastructure and therefore couldn’t rapid charge when out and about, and larger models required bespoke 3-phase chargers to be installed at the depot. With lithium-ion batteries being expensive to buy at the time, Allied and Modec ceased their EV operations by the early 2010s, with Smith relocating its headquarters to the US and soldiering on until 2017.
Tesla negated the expense of large-format lithium-ion cells by using standard “18650” cylindrical cells that were already mass-produced for use in laptop batteries, a strategy that allowed the company to procure enough cells to produce high-capacity battery packs without breaking the bank. Nissan, on the other hand, chose to build their own large-format cell production lines from scratch. The LEAF proved to be an instant success, outselling the Toyota Prius year-on-year in the UK for the first six years from their respective launch dates despite increasing electric competition for the LEAF and decreasing hybrid competition for the Prius over those periods. Other manufacturers partnered with experienced cell manufacturers to create new large cell formats and production lines, and invested heavily in cell chemistry research and development.
As we head into 2020, economies of scale and improvements in the design and production of lithium-ion cells have brought them tantalisingly close to dipping under the $100/kWh barrier, a point at which many experts predict that EVs will become cheaper to buy than ICEs. With autonomous tech coming on leaps and bounds, new ownership and usage models being explored and a number of revolutionary new cell chemistries waiting in the wings, this promises to be an exciting decade for electric transport, with a plethora of choices to suit every need and budget. For the first time since the early 1900s, we have the technology to produce affordable, high-performance electric vehicles; the market demand for manufacturers to do so; and a momentum so strong that no firms clinging onto the final days of oil can stop it.
The next chapter of the electric revolution is very nearly here. Bring it on.